By Hoa Nguyen, Emma C. Ross, Ricardo Cortez, Lisa J. Fauci, and Mimi A.R. Koehl
Organisms often carry captured prey, their own young, or other loads that may hinder their locomotion. While researchers have studied the effects of load carrying on the flight of birds, bats, and insects (all of which operate at high Reynolds numbers where inertial forces predominate), the corresponding consequences on the performance of microscopic organisms (which swim at low Reynolds numbers where viscous forces determine the flow) are poorly understood.
Figure 1. The capture zone around the collar lies one bacterial radius away from the collar. 1a. A capture zone for the model with no attached bacteria. 1b. A capture zone for the model with 18 long-wide bacteria. Note that the capture zone is not a part of the choanoflagellate's structure and does not obstruct the fluid flow. Figure courtesy of the authors.
We studied load carrying at low Reynolds numbers in the context of unicellular choanoflagellates: microscopic protozoans that wave a flagellum to swim and create a water current that transports bacterial prey to a food-capturing collar of microvilli around the flagellum. Our interdisciplinary team of mathematicians and biologists coordinated laboratory experiments, mathematical modeling, and computational simulations to examine the fluid mechanics and feeding performance of unicellular choanoflagellates with and without bacterial prey on their collars [3]. Because the patterns of fluid flow around a choanoflagellate—as well as the mechanisms by which it generates forces for swimming or creates currents—all operate in a viscous-dominated regime, we used the method of regularized Stokeslets [1] to model the hydrodynamics of a swimming choanoflagellate with bacterial prey on its collar. Doing so revealed that swimming speed decreases as the number of prey increases. The presence of bacteria on the collar also reduces the flux of water into a choanoflagellate’s capture zone, and feeding efficiency—a measure that reflects the amount of required work to capture prey, or (in our case) the flux per flagellar cycle divided by the work per cycle—decreases more when prey are in the plane of flagellar beating or near microvillar tips than when they are located elsewhere.
Our study offers insights into the open question of whether changes in the bacterial load on a choanoflagellate’s collar affect its swimming and feeding performance. When combined with experiments, our modeling approach allows us to hold constant morphology and flagellar kinematics in order to isolate and explore the effects of bacteria on the collar. While differences in flagellum or collar length between individual choanoflagellates can significantly impact their relative swimming speeds [2], we find that an individual of any given morphology slows down as it accumulates bacteria on its collar. In our choanoflagellate model, we maintained constant collar and flagellar lengths and controlled the sizes and locations of bacteria on the collar. Specifically, we modeled three sizes of bacteria: long-wide with a length of 2 micrometers (μm) and a radius of 0.67 μm; long-slender with a length of 2 μm and a radius of 0.335 μm; and short-slender with a length of 1 μm and a radius of 0.335 μm. We ran five simulations with different random placements of bacteria on the collar for several scenarios: two, five, seven, or 18 bacteria of each size. Both laboratory measurements and model calculations of the swimming speeds of Salpingoeca rosetta—a particular species of choanoflagellate—demonstrate that bacteria on the collar slow its movement through water.
We quantify the feeding performance via the flux of prey-carrying water into a capture zone — that is, the volume of water that flows into a defined region throughout one flagellar beat cycle. In order for a choanoflagellate to catch a bacterium, the bacterium must contact the collar. We therefore identify the capture zone as the surface around the collar that lies one bacterial radius outside of the collar (see Figure 1). In the absence of caught bacteria, this capture zone is a portion of a conical surface (see Figure 1a). But when bacteria attach to the collar, we remove the regions of the surface that are blocked by the bacteria from the flux calculation (see Figure 1b). We then compute the inward flux of fluid during one flagellar beat cycle as
\[Q=\rho\int^T_0\int_{\mathcal{S}, \mathbf{v}\cdot\mathbf{n}>0}\mathbf{v}\cdot\mathbf{n}\;d\sigma dt.\]
Here, \(\rho\) is the density of water, \(T\) is the duration of a flagellar beat, \(\mathcal{S}\) is the surface of the capture zone, \(\mathbf{v}\) is the fluid velocity on the capture zone relative to the cell, and \(\mathbf{n}\) is the unit inward normal to the capture zone. Due to the different radii of the bacteria types, the capture zone is 0.67 μm away from the collar for the simulations with long-wide bacteria and 0.335 μm away for those with long-slender or short-slender bacteria.
Figure 2. Streamlines and water speeds relative to a simulated choanoflagellate in the flagellar beat plane. The flows are averaged over a flagellar beat cycle. 2a. Choanoflagellate model with five attached bacteria on its collar; the colors depict velocity magnitude. 2b. The zoomed-in region of captured bacterium in 2a. 2c. Choanoflagellate model with no attached bacteria. Figure courtesy of the authors.
Using our model, we calculated the flux for five random placements of varying numbers of bacteria on the collar of a model swimmer. These simulations revealed that bacteria on the collar reduce water flux into the capture zone by redirecting the water flow and occluding parts of the collar. Feeding performance is thus diminished for choanoflagellates that already carry prey, which may affect the rates at which they remove bacteria from patches of water wherein bacteria are dense or sparse. Specifically, the reduction in water flux stems from the redirection of the water flow by the captured bacteria’s three-dimensional structure, as well as the blockage of parts of the collar’s surface area where bacteria are captured (see Figure 2). We discovered that the inward flux decreases most significantly when the choanoflagellate carries larger prey, prey in the plane of flagellar beating, and prey near microvillar tips.
Furthermore, we also constructed both the force and velocity distributions along the flagellum during each phase of the flagellar cycle. We then used these distributions to calculate the mechanical work per flagellar cycle for choanoflagellates that carry differing numbers of bacteria on their collars. Our results indicate that the work does not change significantly based on the bacteria’s location, so feeding efficiency exhibits a similar trend as the inward flux.
Our findings ultimately provide insight into the way in which captured bacteria on a choanoflagellate collar slow swimming speed and reduce the flux of water into the capture zone. As many species of choanoflagellates form multicellular colonies with various configurations, future studies should explore colony formation’s effect on choanoflagellate load carrying.
Hoa Nguyen delivered a minisymposium presentation on this research at the 2023 SIAM Conference on Computational Science and Engineering, which took place earlier this year in Amsterdam, the Netherlands.
References
[1] Cortez, R., Fauci, L., & Medovikov, A. (2005). The method of regularized Stokeslets in three dimensions: Analysis, validation, and application to helical swimming. Phys. Fluids, 17(3), 031504.
[2] Nguyen, H., Koehl, M.A.R., Oakes, C., Bustamante, G., & Fauci, L. (2019). Effects of cell morphology and surface attachment on the hydrodynamic performance of unicellular choanoflagellates. J. Roy. Soc. Interface, 16(150), 20180736.
[3] Nguyen, H., Ross, E., Cortez, R., Fauci, L., & Koehl, M.A.R. (2023). Effects of prey capture on the swimming and feeding performance of choanoflagellates. Flow, 3, E22.
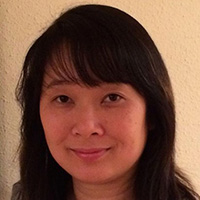 |
Hoa Nguyen is a mathematics professor at Trinity University in San Antonio, Texas, whose research expertise lies in computational fluid dynamics with a specialization in fluid-structure interactions at low Reynolds numbers. She has mentored many undergraduate students from different disciplines while successfully securing internal and external grant funding for both teaching and research. |
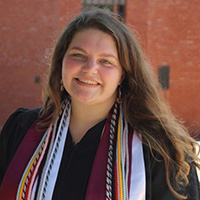 |
Emma C. Ross is a Bridge to a Doctorate Fellow at The Wharton School of the University of Pennsylvania, where she is studying statistics and data science under the supervision of Nandita Mitra. She recently graduated with a Bachelor of Arts in mathematics and Spanish from Trinity University, where she pursued computational fluid dynamics research under the guidance of Hoa Nguyen. |
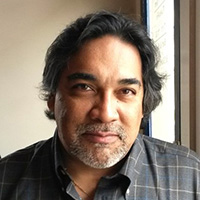 |
Ricardo Cortez is the Pendergraft William Larkin Duren Professor of Mathematics and Director of the Center for Computational Science at Tulane University. He researches fluid dynamics and mathematical modeling, with a focus on the development of computational methods for the simulation of microorganism interactions within a fluid. Cortez is a Fellow of SIAM and the American Mathematical Society, and received the 2012 Blackwell-Tapia Prize for his mentorship and efforts to increase opportunities for students from underrepresented groups in mathematics. |
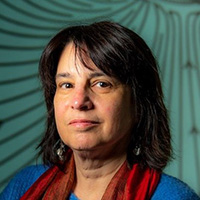 |
Lisa J. Fauci is the Pendergraft Nola Lee Haynes Professor of Mathematics at Tulane University. Her research focuses on biological fluid dynamics, with an emphasis on modeling and simulation to study the basic biophysics of organismal locomotion and reproductive mechanics. Fauci served as president of SIAM from 2019 to 2020 and was elected to the U.S. National Academy of Sciences in 2023. |
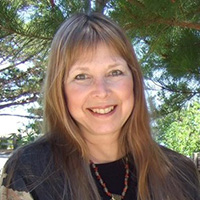 |
Mimi A.R. Koehl is a professor at the University of California, Berkeley, where she performs laboratory and field studies of the biofluid dynamics and biomechanics of the way in which organisms interact with each other and their natural environments. She is a member of the National Academy of Sciences, a MacArthur "Genius Grant" Fellow, and a Fellow of the American Physical Society and the American Academy of Arts and Sciences. Koehl has received various awards, including the John Martin Award from the Association for the Sciences of Limnology and Oceanography, Borelli Award from the American Society of Biomechanics, Rachel Carson Lecture from the American Geophysical Union, and Muybridge Award from the International Society of Biomechanics. |