By Karthika Swamy Cohen
Harmful algal blooms (HABs) occur when colonies of phytoplankton grow at a rapid rate in the ocean or in fresh water bodies, releasing toxic material harmful to other organisms. HABs have caused millions of dollars in economic damage to farmed animals and have untold ecological impacts on marine life and resources such as drinking water.
Phytoplankton are known to contribute 50 to 85 percent of the oxygen in Earth’s atmosphere. When they form HABs, they prevent light from entering the ocean water, causing massive fish kills. The aggregation behavior of these simple organisms is poorly understood, which makes it hard to predict and control HABs.
Heterosigma Akashiwo, a species of phytoplankton, causes harmful algal blooms in the ocean and fresh water.
At the
SIAM Conference on Mathematics of Planet Earth held earlier this month in Philadelphia, Pa., Nicholas Russell of the University of Delaware described agent-based mathematical models to predict and analyze when phytoplankton cause algal blooms. Russel studies the behavior of the alga, Heterosigma Akashiwo – a phytoplankton species that causes HABs. Understanding these behaviors can help determine how plankton signal each other via photosynthesis and direct chemical signaling.
Russell and his team consider chemotaxis, phototaxis, and other possible phenomena influencing the behavior of Heterosigma Akashiwo.
In lab experiments, Russell and his team observe these organisms in a tank fitted with equipment that takes pictures of the algal species every five seconds. Changing the light intensity allows researchers to study the effect of light on movement and thus analyze the influence of phototaxis.
The organisms are seen to form aggregations almost immediately in water – this is even in the absence of any turbulence flow. This movement is seen to happen in the absence of light, so researchers attribute it to a form of chemotaxis. In addition to chemical signaling, the depth of the water column seems to have an impact: increasing the water’s depth results in a different form of aggregation. Photosynthesis also plays a big role and there is a possibility of self-induced hydrodynamics among plankton.
The movement exhibited by the algae is “run and tumble” – the organisms move straight and then eventually flip. This movement is influenced by the gradient of the chemical field. While the direction of the flip is seen to be random, the timing of the flip is not. Flipping rate is based on whether the algae are moving towards a chemical gradient or not. They are less likely to flip if moving towards a chemical gradient and are more likely to flip if moving away.
Russell's computational model incorporates movement phototaxis, chemotaxis, and the fluid dynamics of plankton.
Russell’s team used several probability density functions to describe various facets of the plankton’s run-and-tumble movement. Their model accounts for the velocity of flipping rate, the swimming rate, and the diffusion and decay rates – the rates at which the chemical field diffuses and decays.
As the chemical field develops and run-and-tumble movement progresses, the algae are seen to form elongated axisymmetric aggregations. Russell uses this simple model of chemotaxis and run-and-tumble to create a computational model.
The researchers use a blob detection code in Python—after gray-scaling the images from experimental observations—to count the number of aggregations. They average results from ten repetitive experiments to account for stochasticity and probability. The team sees a large number of aggregations in the beginning, which then asymptote to a certain value.
They conclude that increased flipping rate leads to more aggregations; a higher chemical decay rate also results in more aggregations, while increase in the chemical diffusion rate leads to less aggregation.
Finally, the researchers extrapolate their discrete agent-based model to a continuum model by using an evolution equation. Since the two-dimensional model can get pretty complicated, they chose to go with a one-dimensional model with a coupled set of partial differential equations, and derived steady state results for the chemotaxis model in this case.
The team concludes that chemotaxis plays a very important role in aggregations even in the absence of turbulent flow, and aggregations occur even without external fluid flow.
Future work would involve understanding the stability of aggregations and exit time problems, and incorporating density, photosynthesis, vertical migration, optical attenuation, fluid motion, and the effect of toxins into the model.
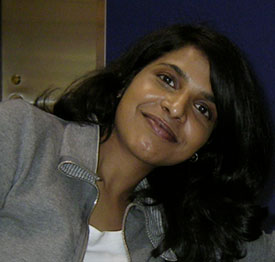 |
Karthika Swamy Cohen is the managing editor of SIAM News.
|