By Lina Sorg
Most people can entrain their circadian rhythms with the 24-hour light-dark cycle. However, certain individuals are unable to do so due to disease or societal obligations, such as an overnight job schedule. As a result, their circadian phases are misaligned. During a minisymposium at the 2022 SIAM Conference on the Life Sciences, which is currently taking place in Pittsburgh, Pa., in conjunction with the 2022 SIAM Annual Meeting, Casey Diekman of the New Jersey Institute of Technology used computational and mathematical methods to explore circadian entrainment in the context of three different scenarios. Specifically, he seeks to better understand management strategies that minimize misalignment in non-24-hour sleep-wake disorder (N24), shift work, and social jet lag.
The subsequent three questions shape the direction of his efforts:
- N24: How does appropriately timed bright light therapy induce entrainment?
- Shift work: Why are the re-entrainment times that follow transitions between day and night shifts asymmetric?
- Social jet lag: What sleep and wake onset times minimize misalignment for teenagers who engage in compensatory catch-up sleep on the weekend?
To answer these questions, Diekman engages one-dimensional entrainment maps—oriented on the well-established Forger-Jewett-Kronauer (FJK) model of the human circadian pacemaker—to measure the phase of the entrained rhythm with respect to the daily onset of light. Various researchers have fit, tuned, and validated the FJK model for numerous experiments about circadian rhythm’s response to light pulses and other stimuli.
Diekman defines certain variables as follows: \(C\) is the core body temperature, \(A\) is the auxiliary variable, and \(N\) is the phototransduction pathway through which light drives the circadian system. He also identified several key parameters:
- \(\tau_c\), which determines the period of the oscillator in constant darkness
- \(I\), the intensity of light
- \(p\), the steepness of the light’s dose-response curve.
To compute the entrainment map, Diekman selects a Poincaré section \(\mathcal{P}\) on the entrained limit cycle (the exact location depends on the topic at hand). He assumes that the oscillator has an initial condition that lies on the selected section \(\mathcal{P}\). The map’s key variable is \(x\): the number of hours since the lights were last turned on. Next, Diekman evolves the trajectory under the flow; when it returns to \(\mathcal{P}\), he records the elapsed time. One can think of the entrainment map as an alternative to the phase response curve in the study of circadian oscillator entrainment.
The entrainment map has certain genetic properties, the most interesting of which is its continuous dependence on the preceding key parameters. After displaying the fixed points on the map, Diekman cobwebbs it to explore its dynamics. If \(\tau_c\) becomes too small or too large, the map loses its fixed points. And when fixed points cease to exist, the solution is no longer entrained. Light intensity also affects the map’s concavity, in that higher levels of intensity makes the map steeper.
Non-24-hour Sleep-wake Disorder
Diekman then applies his entrainment map to the aforementioned three scenarios. He begins with N24, a circadian rhythm sleep disorder wherein an individual’s biological clock fails to synchronize to a 24-hour day. Reduced light sensitivity and variations in the clock’s intrinsic period cause N24, and people who suffer from it experience sleep patterns that are delayed by minutes or even hours on a daily basis. Their circadian clocks are thus free running, meaning that their sleep periods go all the way around the 24-hour clock.
Diekman presents several simulations of N24 in which the intrinsic period of the clock is either faster or slower than normal. He also varies the parameter that affects the steepness of the dose response curve. “When varying either of these parameters alone, we still have stable fixed points for the map, indicating that we still have entrainment” Diekman said. “But if I vary both of these parameters, we can lose our stable fixed points. In the simulations of the full model over many days, you can see that we’re not entrained to a 24-hour clock.”
Diekman uses the map to induce entrainment in a slow and fast clock via bright light therapy (see Figure 1). First, he simulates the application of bright light therapy in the morning—the typical recommendation for N24—for a slow clock. He models a light box with 10,000 lux (a unit that measures one lumen per square meter) over the course of one hour. “This does induce a stable fixed point on the map,” Diekman said. “The entrained phase of that stable fixed point is appropriate — within a couple hours of what someone with a normal clock and normal light sensitivity would experience.” The same bright light also induces a fixed point for a fast clock, but the entrained phase is completely misaligned.
Figure 1. Bright light therapy in the morning via one hour of a light box with 10,000 lux.
In contrast, one hour of 10,000 lux bright light therapy in the evening does not induce a stable fixed point for people with a slow clock, but it does induce a stable—and desirable—fixed point for those with a fast clock. “The outcome of this study is that when administering bright light therapy, it is important to have an estimate of the individual’s intrinsic circadian rhythm,” Diekman said.
Shift Work
Next, Diekman explores circadian misalignment in shift workers. Nearly 15 million Americans work a permanent night shift or rotate in and out of night shifts. Night shift work is associated with a variety of negative health outcomes, including increased instances of cardiovascular disease; gastrointestinal, metabolic, and reproductive dysfunction; cancer; and obesity. Diekman looks specifically at the rotation from one shift to another (i.e., between day and night shifts) and the subsequent re-entrainment process. He considers the following three shifts—each with 17 hours of light exposure—in both a single-lux and more realistic multi-lux scenario:
- Shift 1: Works from 7 a.m. to 3 p.m. and sleeps from 11 p.m. to 6 a.m.
- Shift 2: Works from 3 p.m. to 11 p.m. and sleeps from 12 a.m. to 7 a.m.
- Shift 3: Works from 11 p.m. to 7 a.m. and sleeps from 8 a.m. to 3 p.m.
Diekman finds that transitioning between shifts 1 and 2 is equivalent to entering a new time zone with a one-hour difference; in short, any effects are negligible. But transitioning from shift 1 to shift 3 or vice versa induces quite a bit of jet lag. If the light intensity is low, the re-entrainment time is better when moving from shift 1 to shift 3 instead of from shift 3 to shift 1; this outcome is reversed with higher light intensity.
Figure 2. Social jet lag with recovery sleep on weekends.
Social Jet Lag
Diekman concluded with a quick summary of social jet lag in the context of teenagers, whose tendencies to stay up late and sleep in on weekends cause circadian misalignment. He uses two distinct entrainment maps that correspond to the different types of light-dark exposure on weekdays versus weekends. Diekman seeks to minimize the severe weekday misalignment — especially when young adults are engaging in recovery sleep on the weekends (see Figure 2). “If we look across different amounts of recovery sleep on the weekends, there is always a local minimum in the misalignment at different bedtimes,” Diekman said. “One strategy could involve moving the sleep onset time earlier by half the difference in the photoperiods of the weekday versus weekend light exposure. Moving the wake onset time by that same amount seems consistent with minimizing the amount of circadian misalignment during the weekdays while allowing for some recovery sleep.”
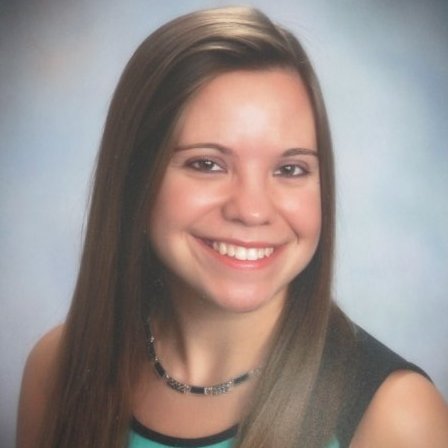
|
Lina Sorg is the managing editor of SIAM News. |