By Jillian Kunze
Climate change has many damaging effects on the planet, including permafrost degradation and ground ice thawing in cold regions. Permafrost—soil that remains continuously frozen year round—stores a large amount of organic carbon. This carbon is released when the soil thaws, furthering climate warming in a dangerous feedback loop. The rate of permafrost degradation varies between different regions, indicating that some additional factors govern the process besides the rise in global temperature.
During a minisymposium presentation at the virtual 2020 SIAM Conference on Mathematics of Planet Earth, which is taking place this week, Dmitry Nicolsky of the University of Alaska Fairbanks described his efforts to investigate the impact of heat flux and water level on permafrost thawing. He presented a model that goes beyond purely temperature-related effects to connect the rate of permafrost degradation with the amount of liquid water in the frozen soil.
Scientists monitor permafrost across the world at particular boreholes—narrow shafts bored into the ground—whose locations are shown on this map. At these deep boreholes, the researchers measure both the temperature profile over soil depth and the heat flux into the ground. These measurements reveal a seasonal trend of heat flux entering the soil during the summer and exiting the soil during the winter, with an additional spike of non-conductive heat flux in the spring. However, it is much more difficult to measure long-term trends in heat flux that occur over many years. Nicolsky thus estimated heat flux by examining the change in temperature profiles of permafrost boreholes over time. He accounted for several complicating factors in his calculations, including the fact that heat flux is sometimes much greater at the surface than underground and can increase when human activity disturbs the surface.
To build his physical model of permafrost degradation, Nicolsky utilized a heat flux equation that incorporated terms for the phase change of water while also accounting for the thermal properties of water and the unfrozen liquid water content. He considered two possible cases of soil type—coarse-grained and fine-grained—which then informed the amount of unfrozen liquid water trapped in the soil. The model operated based on initial conditions for the amount of heat flux into the ground and the starting temperature, and assumed that each soil profile had the same amount of stored energy. No analytical solution exists for the heat flux equations that the model employed, but Nicolsky was able to adapt the classical Neumann solution for this problem. He modeled fine and coarse soil under both natural and disturbed conditions, and used a higher heat flux for the disturbed case.
The results of Nicolsky’s permafrost degradation model under natural surface conditions.
Nicolsky applied his model to predict the results of 100 years of thawing under natural surface conditions, ultimately revealing that the coarse soil’s temperature increased more quickly because heat flux could propagate deeper into the soil. For fine soil under the same conditions, the heat flux seemed to dissipate more. Unsurprisingly, more heat flux was able to penetrate into the ground for both soil types after 500 years, thus melting permafrost at deeper levels than after only 100 years. Based on projections of permafrost thawing over multiple time spans, fine-grained soil did not experience much change at the surface under natural conditions, though some phase changes occurred in the water throughout the column. For the coarse material, much of the heat flux was spent melting the permafrost near the surface. The soil types responded much more similarly to each other under disturbed surface conditions than they did under natural surface conditions.
The results from Nicolsky’s model revealed that the rate of permafrost melting in the layers closest to the surface could be significantly less for fine-grained than coarse-grained soil, as long as all other factors remain the same and the permafrost degradation had just begun. The amount of incoming heat flux has a nonlinear effect on the melting rate, and the difference between the rates for varied soil types is much more drastic for lower values of heat flux. These results could be important for future observations of permafrost thawing and may lead to a better understanding of global warming’s impact on permafrost.
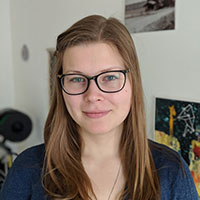
|
Jillian Kunze is the associate editor of SIAM News. |