By Lina Sorg
Adult humans have an inherent circadian rhythm of approximately 24 hours that corresponds to the 24-hour day and revolves in part around available daylight. Dynamic mathematical models measure the effect of light/darkness on the circadian system and the ways in which circadian rhythms and wake time impact objective performance and subjective alertness. Individuals often self-select differing sleep/wake times depending on social preferences or work demands; this is especially true on weekends, when sleep patterns alter from weekdays and varied light exposure impacts circadian oscillators. The tendency of people to sleep at particular times during the 24-hour day is their “chronotype” — a behavioral manifestation of their preferred sleep/wake cycle.
During a minisymposium at the 2018 SIAM Annual Meeting, currently taking place in Portland, Ore., Elizabeth B. Klerman of Harvard Medical School applied the principles of circadian rhythms to human physiology. “We try to do modeling where we can match the physiology to the mathematics,” Klerman, who is also the director of the Analytic Modeling Unit within the Division of Sleep Medicine at Brigham and Women's Hospital, said. “We model sleep and circadian rhythms to identify key elements of the system and organize our understanding of it.” Models can also explain contradictory results; generate testable hypotheses; design experiments; and test or predict outcomes of potential schedules or conditions without having to carry out each possible one, which is both costly and time-consuming.
Structure of the circadian rhythm paradigm.
Through her research, Klerman aims to simulate different sleep-wake conditions to predict instances of poor performance and alertness, adjust the timing of lighting within schedules via control theory, and explain the resulting data. She began with a brief overview of the factors that influence overall physiology, including environment and behavior; neurobehavioral issues like sleep-wake propensity, alertness, cognitive performance, and vigilance; and physiological conditions like melatonin and body temperature. “We know the physiology, so we need to be able to put it into a model,” Klerman said.
She presented a set of Jewett-Kronauer-based circadian and performance models that account for increasing numbers of physiological factors as researchers better understand sleep physiology. Klerman then introduced a previous study that simulated proposed sleep schedules for NASA astronauts while aboard space shuttles. “The original launch time felt like 2 or 3 a.m., not the time you’d expect astronauts or crew to be wide awake,” she said. As a result, mathematician Dennis Dean changed the timing of light levels so the launch time was equivalent to several hours earlier. Dean also optimized schedule building blocks to better understand phase shifts. “He showed that for the equivalent of a 12-hour shift, it takes about a week for that 12-hour phase shift if you don’t have countermeasures,” Klerman said. “But if you apply light at the right time and start shifting circadian rhythms more quickly, your performance throughout the day shifts the baseline much faster.”
Comparison of initial and adapted, optimized proposed sleep schedules for NASA astronauts while aboard space shuttles.
She then discussed another example in which mathematician Andrew Phillips sought to include circadian rhythms in a sleep-wake model. He experimented with the addition of light, naps, and caffeine as countermeasures, each of which helps the subject perform better during an ultimate critical event. “If you add them all in, the model predicts that you’ll do just fine,” Klerman said. If someone needs to do perform a task at a particular time, it can offer a general idea of whether or not he/she will perform well.
Another sleep-wake project modeled the rigorous schedules of medical residents, which typically display as double-plotted raster plots. Researchers proposed two new types of schedules—including a rapid cycle rotation—and compared them to existing Q3 and Q4 schedules, in which residents work every third or fourth night and thus get two or three consecutive nights of sleep respectively. They compared objective performance and subjective alertness in both individual residents and the collective medical team. Analysis revealed that while the new schedule types yielded no behavior changes during the day, they improved operation of the residents at night; the original Q3 and Q4 schedules placed fewer people on call at night, all of whom were more tired.
The inconsistent sleep schedules of undergraduate students also lend themselves well to sleep-wake modeling. College students actively shift their sleep-wake schedules by frequently exposing themselves to artificial light well after it’s dark outside and lower levels of natural light when spending the day indoors. This misalignment of biological and social time is called social jetlag, and is common in students and those keeping drastically different sleep-wake schedules on weekdays versus weekends. “There is increasing evidence that social jetlag is bad for your health,” Klerman said.
In conclusion, researchers can use mathematical modeling to explore multiple interactions, inputs (i.e., caffeine, napping, and light exposure), and outputs related to circadian rhythms and sleep (i.e., chronotype, sleep/wake timing, lighting, and imposed or self-selected sleep schedules). Better understanding the effects of these factors can help researchers interpret sleep-related physiological observations and make suggestions for individual and group work schedules to improve alertness, performance, and sleep quality.
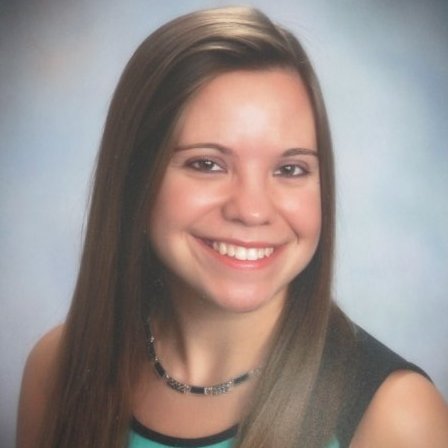 |
Lina Sorg is the associate editor of SIAM News. |