The Impact of Carbon Dioxide Sequestration on Shallow Groundwater
By Hari Viswanathan, Zhenxue Dai, Christina Lopano, Elizabeth Keating, J. Alexandra Hakala, George D. Guthrie, and Rajesh Pawar
Numerous comprehensive analyses have concluded that recent increases in average global temperature are likely a result of increased concentrations of carbon dioxide and other greenhouse gases in the atmosphere [2]. Storing CO2 in geologic formations is one approach for mitigating excess anthropogenic CO2 from centralized sources, such as coal-fired power plants [1]. One concern about geologic sequestration is the possibility that CO2 will leak from the sequestration reservoir, affecting overlying aquifers utilized for drinking water. For this reason, groundwater protection is of vital importance to carbon capture and storage (CCS) projects.
Migration of CO2 from deep storage formations into shallow drinking water aquifers is a possible system failure of geologic CO2 sequestration. Although CO2 is not toxic in low concentrations, increased concentrations in the shallow subsurface could cause a decrease in pH levels. This, in turn, could result in the mobilization of trace metals from aquifer minerals and subsequently increasing levels of naturally occurring contaminants, such as arsenic, lead, and uranium [4]. Subsurface injection of large amounts of CO2 could also displace large amounts of brine, which might already contain elevated concentrations of toxic metals [3]. Trace metal concentrations could exceed those specified in the National Primary Drinking Water Standards of the Environmental Protection Agency. Risk assessment for CCS requires quantitative information about the potential impact of CO2 and brine leakage on overlying aquifers; this impact, however, is largely unknown.
In the study described here, a CO2 sequestration site in Chimayo, New Mexico, was examined for site-specific impacts of shallow groundwater interacting with CO2 from the deep storage formations. The work focuses on As, which is regulated by EPA under the Safe Drinking Water Act and which is present in some wells in the Chimayo area at concentrations above those given in the Maximum Contaminant Level (MCL). Statistical analysis of existing Chimayo groundwater data indicates that the presence of As is strongly correlated with that of trace metals U and Pb, indicating that the source of all three may be the same deep subsurface water. Batch experiments and material characterizations, as by X-ray diffraction, scanning electron microscopy, and synchrotron micro X-ray fluorescence (\(\mu\)-XRF), were used to identify As association with iron-rich phases, such as clays or oxides, in the Chimayo sediments as the major factor controlling the fate of As in the subsurface. Figure 1 shows \(\mu\)-XRF results in which trace metals, including U and As, were correlated with Fe in most of the shallow Chimayo sediments.
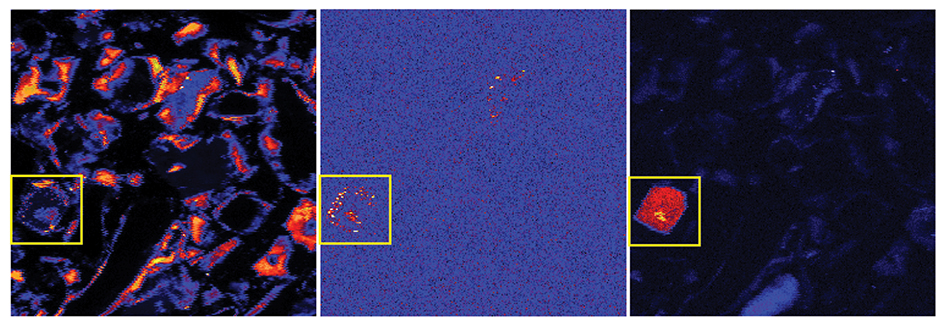
Figure 1. Selected element distribution maps (iron, left; arsenic, center; uranium, right) for sample Roadcut Lithosome A illustrating that As and U trend with Fe (since they appear to be associated with iron-bearing minerals in the clay) in this portion of the Chimayo sediment. A yellow box has been drawn around an area of interest in these false-color maps (in which warmer colors correspond to higher concentrations of each element). Maps were collected at 19 keV with a step size of 2 µm. Each map area is 500 µm x 500 µm.
|
Batch laboratory experiments with Chimayo sediments and groundwater show that pH decreases as CO2 is introduced into the system and buffered by calcite (Figure 2). The introduction of CO2 causes an immediate increase in As concentration, which then decreases over time. A geochemical model developed to simulate these batch experiments successfully predicted the drop in pH once CO2 was introduced into the experiment. In the model, adsorption of As to illite, kaolinite, and smectite through surface complexation proved to be the key reactions in simulating the drop in As concentration as a function of time in the batch experiments. Based on analysis of the model, kaolinite precipitation is anticipated to occur during the experiment; this allows for the formation of additional adsorption sites with time, resulting in the slow decrease in As concentration (Figure 2). This mechanism can be viewed as trace metal “scavenging” resulting from adsorption-caused secondary mineral precipitation.
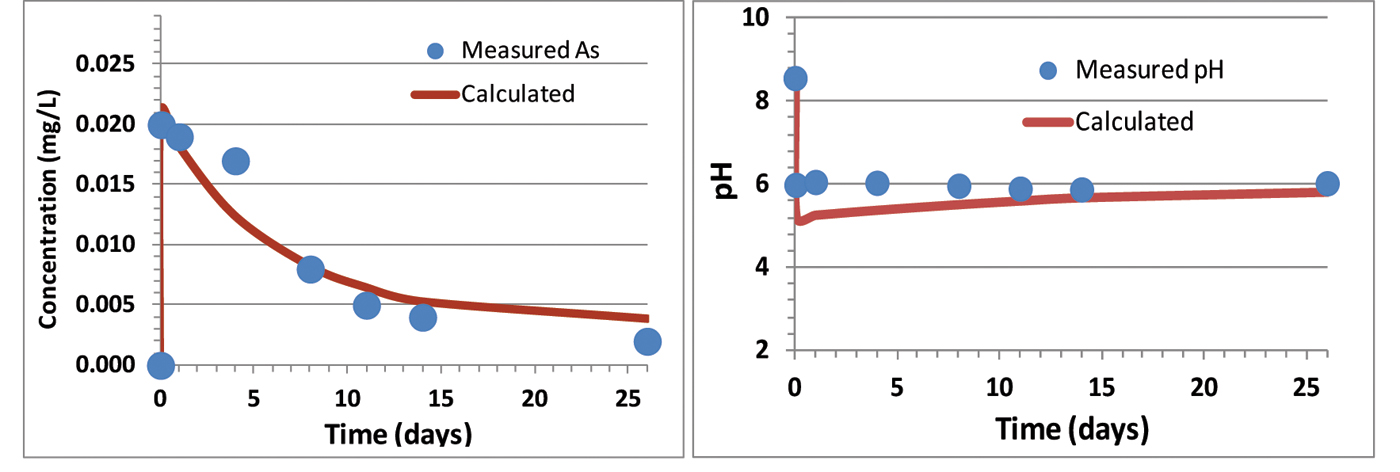
Figure 2. Inverse modeling results of the batch experiment with sample Roadcut Lithosome B.
|
In summary, the data show that arsenic upwells from the deep aquifer with CO2 and then (under the oxidizing conditions of the aquifer) adsorbs to iron-bearing minerals (e.g., clays) at the Chimayo site, retarding transport of As in the shallow groundwater. Other trace elements, such as U or Pb, may be controlled by completely different processes. The risk assessment of each trace element of concern will thus require fairly complex analysis to determine the key parameters affecting its fate at the site. A combination of laboratory and field observations will have to be joined with geochemical modeling to identify the key parameters of interest. Unlike major ions, trace elements exist at concentrations so low that processes like scavenging by secondary minerals can greatly affect their fate and transport in the subsurface.
A full description of this work has been submitted to the International Journal of Greenhouse Gas Control.
Acknowledgments: The authors acknowledge Department of Energy Award DE–FE0001112 Headwaters Project and Zero Emission Research and Technology II programs for funding this work.
References
[1] S. Bachu, Sequestration of CO2 in geological media: Criteria and approach for site selection in response to climate change, Energy Convers. Manage., 41 (2000), 953–970.
[2] IPCC, Climate Change 2007: The Physical Sciences Basis, a contribution of Working Group I to the fourth assessment report of the Intergovernmental Panel on Climate Change, approved at the 10th Session of Working Group I of the IPCC, Paris, 2007.
[3] P.H. Stauffer, G.N. Keating, R.S. Middleton, H.S. Viswanathan, K.A. Berchtold, R.P. Singh, R.J. Pawar, and A. Mancino, Greening coal: Breakthroughs and challenges in carbon capture and storage, Environ. Sci. Tech., 45 (2011), 8597–8604.
[4] L. Zheng, J.A. Apps, Y. Zhang, T. Xu, and J.T. Birkholzer, On mobilization of lead and arsenic in groundwater in response to CO2 leakage from deep geological storage, Chem. Geol., 268:3–4 (2009), 281–297.
Hari Viswanathan, Zhenxue Dai, Elizabeth Keating, and Rajesh Pawar are researchers in the Earth and Environmental Sciences Division at Los Alamos National Laboratory. Christina Lopano, Alexandra Hakala, and George D. Guthrie are researchers in the Geological and Environmental Science Focus Area at the National Energy and Technology Laboratory.